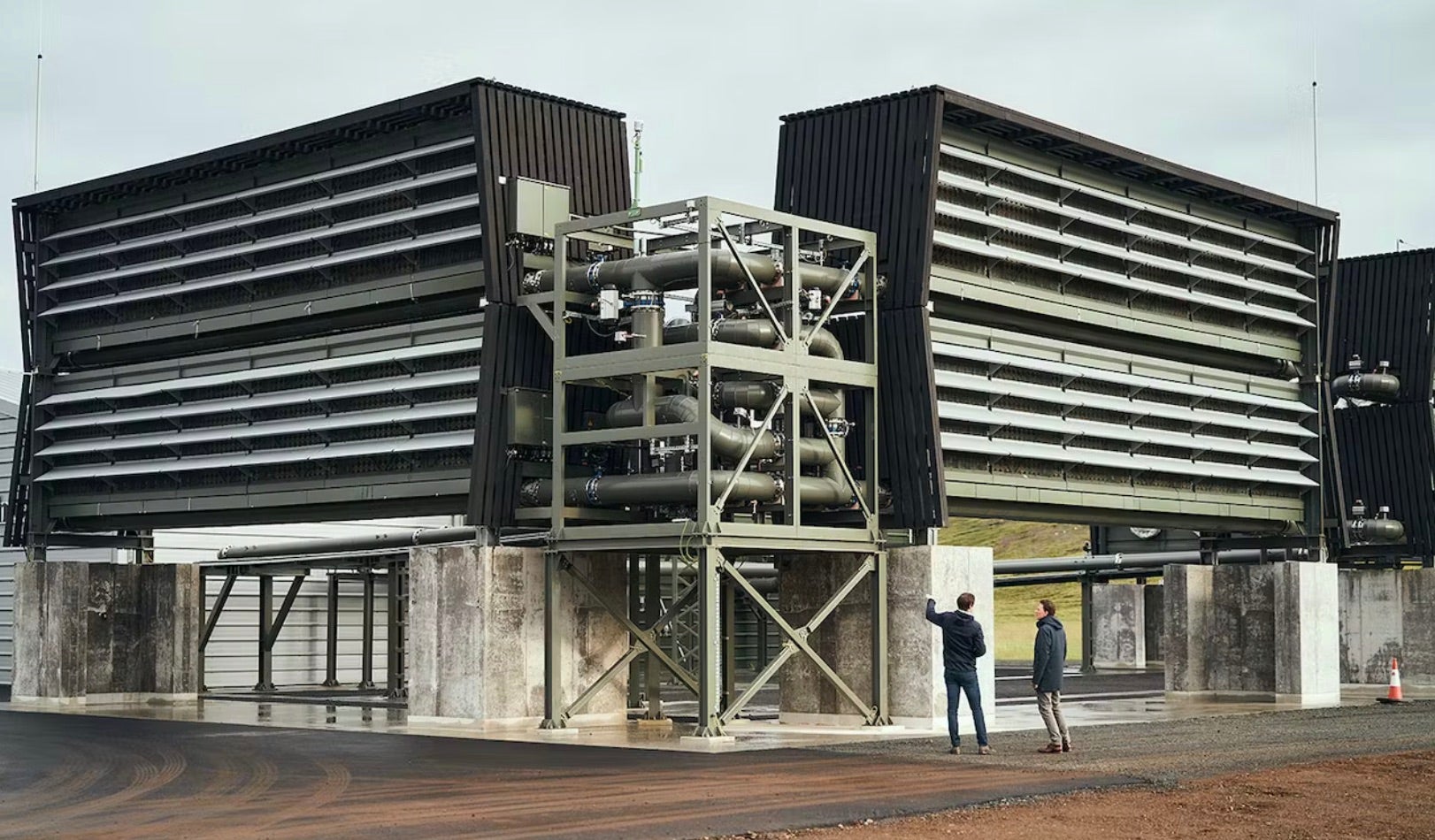
According to the Intergovernmental Panel on Climate Change’s (IPCC) latest report, carbon removal, or carbon dioxide (CO₂) removal (CDR), will be essential to keep global warming to a manageable level. Exactly how much CO₂ will need to be removed depends on how rapidly, and by how much, emissions can be reduced across sectors, but the IPCC estimates that by 2050, an eye-watering 5–16 gigatonnes (Gt) of CO₂ will have to be sucked out of the air annually across the planet.
The US-based environmental think tank RMI recently published a pioneering Applied Innovation Roadmap (AIR) for the carbon removal industry, providing a perspective on how best to advance the technical readiness of 32 known CDR approaches this decade. The road map aims to provide transparency about the risks and opportunities of all known CDR approaches, and offers visibility and guidance on the industry’s research, development and demonstration requirements.
Energy Monitor sat down with Rudy Kahsar, manager of RMI’s carbon removal team, to find out about the industry’s development thus far.
Can you give us some background on the research: why did you do it, what did it entail and what purpose is it meant to serve?
At RMI we try to be unbiased in our support of the energy transition, and within our CDR team what we really care about most is seeing CDR succeed. That might mean helping companies understand what barriers they need to overcome, or helping funders like governments and philanthropies understand what they need to fund and how much they need to fund it. What we really tried to do with the AIR [is] not just come up with a menu of things that need to be funded but focus that menu on critical pathway activities.
We don’t have time to just do open-ended research for 30 years. We need to be very focused on the key things that are critical to getting to the next stage. The AIR covers all known approaches for carbon removal, so we’ve done it in an exhaustive way.
What did you find out about the general state of development of the CDR industry? Is it behind or ahead of schedule in its bid to meet the IPCC’s demand of 5–16Gt of CO2 removal a year by 2050?
One of our main takeaways was the realisation of just how much the industry has to scale for us to hit our goals. The 10Gt a year in 2050 that many say is required would make CDR a bigger industry in tonnage than any industry that exists on Earth. That’s from slightly over ten million tonnes today – and we want to create that in just 27 years. That’s a challenge!
But, saying that, another takeaway I had was, ‘wow, a lot of these approaches are ready to be deployed; you could build a demo of most of these next week’. I wasn’t really expecting that because it’s such an early-stage field.
You categorise the different CDR approaches as ‘biogenic’, ‘synthetic’ and ‘geochemical’. Can you explain those definitions? Are any of the categories more advanced than others?
The logic is that if we’re thinking about achieving deployment long term, there are key limiting inputs behind carbon removal. Those three inputs are: biomass or biogenic materials, alkaline materials or rocks, and low-carbon energy.
For example, there are a lot of different ways of doing biogenic CDR: you can bury biomass, you can burn it in a BECCS [bioenergy with carbon capture and storage] plant to make electricity and CO₂, [or] you can do pyrolysis to make biochar [to spread on soil where it can sequester carbon]. But in all of those cases, you’re drawing on the same supply of biomass, whether it’s cultivated biomass or waste biomass, and there is a cap on how much the world has. Right now those approaches can all develop in parallel but long term they’re going to compete with each other for their primary feedstock.
It’s similar with the synthetic approaches that rely on low-carbon energy. Whether you’re doing DAC or direct ocean capture (DOC), etc, you’re going to be competing with the wider energy transition for low-carbon energy. Same thing with alkaline minerals [for geochemical CDR, or enhanced rock weathering]: what volume of alkaline minerals do we have? We can put them on beaches, we can put them on fields, but the point is, [there are] limits in terms of the amount of rocks we have available.
In terms of who has the lead, I think the biogenic space currently and it has an easy path to staying a leader in the near term, because forestry is already doing ten million tonnes a year of carbon removals. But it requires serious improvements on measuring and verification. Similarly, things like biochar are already being done and can scale pretty quickly in the near term – we think it’s pretty safe, [and] we think you can do it to a decent scale.
Something like DAC is starting from a very small base; DOC is even smaller. But by 2050 there could be a gigatonne of DAC, which could scale to ten or 15Gt a year by 2100. [In contrast,] biogenic CDR is going to be capped by the limited amounts of biomass and so it’s not really going to grow as a proportion of total removals in the second half of the century.
The geochemical approaches are much more of an open question. On the one hand, in practice, they’re very easy to deploy: you just grind up rocks and put them on a field. But in another sense they’re a big question mark, because there are potential environmental impacts that need to be addressed [some rocks contain nickel and cobalt which can be environmental pollutants]. There are also questions over measurement: at this point, we don’t have enough data to know very accurately how much carbon removal you can claim per tonne of rock spread. Geochemical removal would also probably require the most space: between 2050 and 2100, it would require 3Gt of alkaline material deployed globally each year over a land mass the size of India. When you start thinking about that kind of scale, you would need an incredible amount of community engagement as well as a huge workforce.
Which individual CDR approaches or technologies currently lead the pack?
The living biomass approaches are already being deployed – but they need to scale [further]. Then there’s approaches like using timber for building materials; there are already people building skyscrapers out of timber in Europe. The challenge there is that there’s a huge question over whether the life cycle assessment (LCA) is in fact negative and you’re actually removing emissions. The same could be said about BECCS. The point is that there are a number of approaches that are already nominally at commercial scale but still have questions around them.
Maybe the most compelling approach, to answer your question, is biomass direct storage. This is as simple as it comes. You literally take biomass, typically waste biomass, and you either sink it in an anoxic environment, you bury it in a hole, you put it in a warehouse, or you put it in say the Antarctic, where it’s not going to decompose [and emit major greenhouse gases such as CO₂, CH4 and N₂O]. There’s a company called Rewind Earth from Israel, and they’re looking at sinking waste biomass from Turkey in the Black Sea, which is a 2,000m-deep anoxic environment. There’s another one called Kodama in the US, which is being paid by the Forest Service to cut down dead beetle-killed trees in the Sierra Nevadas in California and bury them in the Nevada desert.
The compelling element with this approach is its simplicity; I expect a lot of activity from it in the near term.
Are there any approaches that are not very advanced but have great long-term promise?
I would keep an eye on micro-algae sinking and macro-algae sinking. People have talked about ocean fertilisation [adding nutrients to the upper layers of the ocean to stimulate phytoplankton activity; photosynthesis absorbs CO₂] for years. But if you can figure out how you create plankton blooms and then sink them so you can verifiably remove that CO₂, the potential is tremendous. I don’t think we’re going to figure it out in the next ten or 15 years, but [we expect] these approaches [to] begin to grow in the 2040s and in the second half of the century they could supply many gigatonnes of carbon removals.
Another one is the electrochemical ocean approach, which we call ‘indirect water capture‘, [this involves capturing carbon stored in water]. This has a lot of potential because you’ve taken out the contact with the air you have in a DAC plant. There’s still a lot to figure out in terms of how you move that much water to strip the CO₂ out of it. But if that can be figured out, you’ve eliminated the need to install massive fans across huge land masses. You can even integrate it into wastewater treatment plants, or maybe do it on barges or underwater. I think it could potentially overtake DAC in the future.
There have been some controversies recently around several CDR approaches including afforestation, seaweed farming, enhanced rock weathering, BECCS and soil carbon sequestration. Are you concerned about the safety of CDR if deployed rapidly and at scale? Does the AIR help identify potentially dangerous or ineffective approaches?
That was our goal. We include a “fail fast” perspective: if an approach is not going to work, we want to stop funding it. The way we do that in the AIR is with “stage gates”: each of our approaches has a road map, and in each of the road maps there are stage gates that an approach needs to clear before it reaches demonstration scale. These stage gates are: it’s safe for the environment, it actually produces removals, it’s been measured and verified at this scale. These are repeated at demonstration scale: have we confirmed that the environmental impacts are manageable? Have we confirmed that the LCA is properly negative? Have we confirmed that the cost is within line of what’s viable? If not, do not proceed – or proceed at your own risk.
Across these approaches, there are many environmental questions, questions about cost and durability. No CDR approach is perfect in every area. So, there’s going to be a need for a portfolio approach, to weed out the bad ones as we go along. At the scale we’re going to need to do this, there are going to be some negative impacts at some point, but that is still far better than the catastrophic impacts of climate change that are heading our way.
Is there anything you can tell us about which approaches are proving the most effective and scalable forms of CO₂ storage?
In most biogenic and geochemical approaches, the storage is in the biomass or materials themselves. But in specific CO₂ storage, there are three approaches: conventional CO₂ storage, which is in things like saline aquifers and salt caverns; in-situ mineralisation, which is what companies like Carbfix are doing; and ex-situ mineralisation, which is mineralising your CO₂ stream with rocks that have already been dug up. The global potential for these is just unbelievable, it’s more potential storage than we could ever use, or will ever need. So that’s good news. That doesn’t mean it’s all accessible or financially viable to develop. That’s the next question: what part of it is financially viable and what can we actually get permits to do?
Most of the storage in the near term will be conventional storage – you’re basically putting CO₂ in gas form into a salt cavern or some kind of underground structure – because that’s what the oil and gas industry is used to doing. There are a couple of salt caverns in Texas that have been bought up by Occidental [Petroleum Corporation]; there’s ideas for sequestering CO₂ in the North Sea – both from CDR and CCS; and Carbfix plans to do three million tonnes of carbon mineralisation a year by 2030. So, there’s lots of cool stuff going on.