Nuclear Fusion Power the Race to Net Zero
“The old joke is that nuclear fusion is 30 years away and always will be,” quips Greg De Temmerman, managing director of Paris-based energy think tank Zenon Research. In fact, the joke has become so hackneyed over the decades it has been banned by editors at the Economist. “But more seriously, many things are happening right now in the field,” De Temmerman adds.
Jokes aside, they are. Earlier this month, China’s Experimental Advanced Superconducting Tokamak (EAST) reactor eclipsed previous records by sustaining a high plasma temperature for minutes (1,056 seconds). It reached two milestones: a one-million-ampere current and a 1,000-second duration 100-million-degree temperature (that is five times hotter than the sun). Back in August 2021, the National Ignition Facility at the US’s Lawrence Livermore National Laboratory also heralded a breakthrough using a different approach: it deployed powerful lasers to start a fusion reaction that generated 1.3 megajoules (MJ). That is only about 3% of the energy contained in 1kg of crude oil, but it was another milestone. Meanwhile, private nuclear fusion projects have been booming in recent years.
Nuclear fusion has long been considered the energy of the future: a supposedly infinite source of power that does not produce CO2 emissions. However, after decades of research, it is still to deliver on its potential. How much closer do these new breakthroughs bring us? What role could nuclear fusion play in the world’s race to hit net zero by 2050?
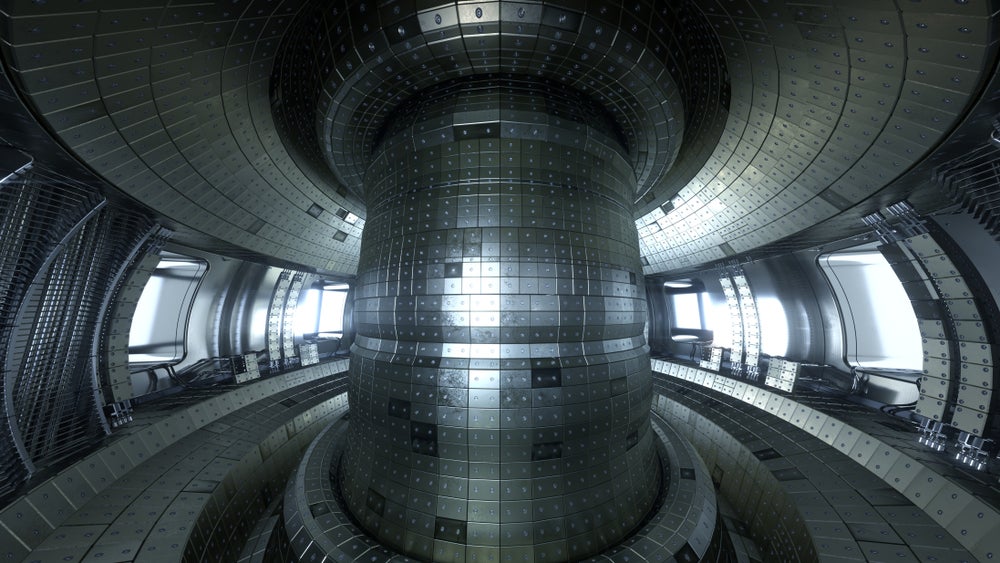
The energy of the future
There are two ways of creating nuclear energy: fission, used in current nuclear power plants, and fusion. In fission, heavy uranium atoms are broken down into smaller atoms to release energy. Fusion is the opposite: small, light atoms are melded together into bigger, heavier ones, as occurs in the plasma core of the sun.
A fusion reactor amplifies power: the reaction must produce more energy than was used to heat up the fuel plasma for energy production to occur. This is known as ‘ignition’ and it has yet to be achieved. The current record dates back to 1997, when the Joint European Torus (JET) in the UK used 23MW to generate 16MW of power by magnetic fusion.
There are two main techniques for creating fusion: magnetic confinement, which uses magnetic and electric fields to heat and squeeze the hydrogen plasma, and inertial confinement, which uses powerful lasers to compress the fuel and initiate the fusion reaction.
How well do you really know your competitors?
Access the most comprehensive Company Profiles on the market, powered by GlobalData. Save hours of research. Gain competitive edge.
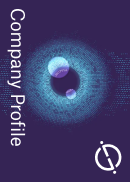
Thank you!
Your download email will arrive shortly
Not ready to buy yet? Download a free sample
We are confident about the unique quality of our Company Profiles. However, we want you to make the most beneficial decision for your business, so we offer a free sample that you can download by submitting the below form
By GlobalDataHistorically, magnetic fusion has been the method of choice, as the technology for inertial fusion – particularly the lasers – has only recently become available. Inertial fusion also needs to produce much more energy to compensate for the lasers’ gluttonous consumption.
The two largest inertial confinement projects are the NIF in the US and France’s Laser MégaJoule. The 1.3MJ produced by the NIF in August smashed its own 2018 record for energy released from fusion reactions 23 times over. This advance saw 70% of the laser energy put in released as nuclear energy, the closest the inertial technique has come to ignition, and equalling JET’s 1997 record.
The team behind the success have managed a more than 1,000-fold improvement in energy release over the last ten years. Jeremy Chittenden, co-director of the Centre for Inertial Fusion Studies at Imperial College London, declared after the breakthrough: “The pace of improvement in energy output has been rapid, suggesting we may soon reach more energy milestones, such as exceeding the energy input from the lasers used to kick-start the process.” However, De Temmerman cautions, a reactor will have to produce a hundred times the energy it consumes to become economically attractive.
The magnetic confinement approach offers better development prospects. Most of the research focuses on tokamaks, fusion reactors created in the former Soviet Union in the 1960s, where plasma is confined by a strong magnetic field. ITER, a demonstration tokamak involving 35 countries under construction in the south of France, will be the world’s largest fusion reactor. It aims to produce ten times as much power as it consumes by heating the plasma with 50MW to produce 500MW of energy. The first plasma (where the reactor is able to generate a molten mass of electrically charged gas – plasma – inside its core) is forecast for December 2025, with a demonstration of fusion expected in the late 2030s.
“Being a very big international project and an extremely complicated device, ITER has been beset by delays and cost overruns,” says De Temmerman. “The first plasma is not expected before 2026, and delays are set to be announced following the pandemic and other challenges. After ITER, many countries foresee demonstration power plants, which would come online in the 2050s.”
The UK has recently launched the STEP project (Spherical Tokamak for Electricity Production), aiming to develop a reactor that connects to the energy grid in the 2040s. China is developing a programme to develop tritium breeding technology – a core component for future fusion reactors to achieve fuel self-sufficiency – in the 2040s. The EU plans to open another tokamak demonstrator, DEMO, in the 2050s.
Another magnetic confinement configuration called the stellarator, exemplified by Germany’s Wendelstein-7X, is also showing promise. Although lower-performing than a tokamak – a slightly different coil configuration changes the topology of the magnetic field – the stellarator’s intrinsic stability and auspicious recent results make it a serious contender.
Fusion power scales strongly with size, and so the trend has emphasised bigger devices that match the output of fission power plants. However, the development of new technologies, such as high temperature superconducting magnets, and the evolution of different confinement techniques, is changing that paradigm. Many of the recent fusion projects are smaller, with targeted power outputs of 100–200MW or less.
“That shift is in part driven by the strong deployment of renewables, which tend to make large power plants seem less attractive,” says De Temmerman. “There is also the economic argument that smaller units would reduce capital costs, hopefully making the upfront investment easier to handle; the examples of Hinkley Point C or the future EPRs in France show that it is difficult to secure the capital costs.”
This has opened the industry to commercial players, which have fast been climbing aboard in recent years. Most of them envision a fusion reaction in the next ten to 20 years and have together attracted more than $2bn in funding, outpacing the traditional public sector development, according to the Fusion Industry Association. General Fusion has raised $130m; Helion Energy $500m, with another $1.8bn committed based on hitting key milestones; and Commonwealth Fusion Systems has closed a $1.8bn venture round. According to Arthur Turrell, author of The Star Builders: Nuclear Fusion and the Race to Power the Planet, the likes of Jeff Bezos, Peter Thiel, Lockheed Martin, Goldman Sachs, Legal & General and Chevron have all invested.
Nuclear fusion: A role in the race to net zero?
Fusion has a lot going for it as a clean energy source. It releases much more energy than fission and produces no high-level nuclear waste. A fusion reactor would not produce CO2 emissions or run the risk of a nuclear meltdown – if any disturbance occurs, the plasma cools within seconds and the reaction stops. Fusion could produce energy regardless of wind conditions or hours of sunlight, and does not require enriched uranium, which can be repurposed for nuclear weapons. Fusion is a compact energy source, boasting high power production per unit of land area.
[Keep up with Energy Monitor: Subscribe to our weekly newsletter]
“In principle, capacity is limitless, as water is the main fuel,” says Dr Michael Bluck, director of the Centre for Nuclear Engineering at Imperial College London. “However, this is misleading – these reactors require deuterium and tritium (‘DT’, both isotopes of hydrogen) as fuel. Deuterium exists naturally in seawater and is relatively easy to obtain but tritium does not occur naturally in significant quantities. There are sources – some fission reactors produce tritium and Fukushima has plenty stored in tanks at the moment – but to be sustainable, fusion reactors have to produce it themselves from lithium [there is only 20kg of tritium currently available]. There might even be competition for resources between batteries and fusion as a result.”
With respect to cost, some start-ups such as Helion Energy have predicted fusion electricity at a few cents per kilowatt-hour, but De Temmerman deems that overly optimistic. “In the 1960s, nuclear was supposed to become ‘too cheap to meter’, while in practice, the costs of nuclear have increased quite a lot. The first reactors, as for every technology, would certainly be more expensive; the question is how fast the learning rate can be.”
The Intergovernmental Panel on Climate Change's landmark report in 2018 concluded that the world needs to reach net-zero greenhouse gas emissions by 2050 to have a good chance of limiting global warming to 1.5°C. Estimates for when fusion might come into use range from 2030 to 2050, and beyond. “Personally, I would be surprised if there is anything practical – by which I mean economic – before 2040, so fusion's role in net zero by 2050 would be limited,” says Bluck.
Net zero by 2050 assumes emissions are constantly in decline from now until 2050. As it is the total amount of CO2 in the atmosphere that sets the eventual level of warming, it is cumulative emissions that matter. So even if fusion power were viable by 2030, it would likely be too late to make much of a difference.
“Fusion has a very marginal role to play [in the net-zero race],” says De Temmerman. “Even if the private projects do succeed in achieving a fusion reactor within ten to 20 years, it is important to realise that building one reactor does not do much for the energy transition. The global installed electricity production capacity is currently over 7,000GW. To get to even a few per cent of that will require building a large number of reactors and that will take time."
Reaching ignition is just one of the many challenges on fusion’s horizon. A commercial reactor will have to solve a variety of daunting engineering problems such as extracting the heat energy and finding materials that withstand the relentless bombardment the reactor chamber will receive over its lifespan – and, as Bluck mentioned, fusion reactors must also be self-sufficient in tritium. This requires surrounding the reactor chamber with lithium because its atoms are converted to tritium when struck during fusion – and this process has yet to be demonstrated at scale. “These are very, very big and inter-related challenges. It is [also] entirely unclear how laser inertial confinement can be done repeatedly and energy extracted,” says Bluck.
A post-carbon energy
The most likely role for fusion is as an energy source in a post-carbon society, but, according to both De Temmerman and Bluck, it is worth funding for that reason alone. The world is likely to need fusion well beyond 2050: as a source of large-scale power to extract existing CO2 out of the atmosphere, and as the only feasible energy source with which to explore the further realms of space.
For De Timmerman, fusion has many advantages but its development should not distract from the very pressing objective of decarbonising as fast as possible. “That said, the amounts currently invested, a few billion dollars a year, are totally negligible compared with the trillions needed for the transition, so it is unlikely that the development of fusion will affect anything for now. One should just be careful to not oversell it as the solution,” he cautions.
For Bluck, if the world can come up with grid-scale storage technology then renewables will be the “horse to back” and there will be little need for fusion – or fission for that matter. “But we don’t have that and it is really not clear if we ever will,” he adds. The International Energy Agency forecasts that storage must increase from the 18GW of capacity in operation today to 590GW in 2030 and 3,100GW in 2050.
A more pertinent question is whether to prioritise fission over fusion. Plainly fission has its issues, such as accidents and waste, but Bluck believes funding R&D in the area would go a long way to addressing them. “At least we know how to build them and they would certainly make some contribution to the net-zero target,” he argues. The Netherlands recently unveiled plans to return to nuclear power.
“Small fusion has received some significant funding from private investors, often billionaires with plenty of cash,” Bluck adds. “Maybe that is where much of the funding should go for now. It is high risk and return – arguably vanity projects – but who knows, maybe this is the drive that will get fusion over the line.”
So, for the next 30 years, watch this space.