Progress of Small Modular Reactors (SMRs)
The growing urgency of the climate crisis and, more recently, the energy crisis has reawakened global interest in nuclear energy. Even the likes of Bill Gates and Elon Musk have waded into the debate to petition for a more prominent role for nuclear power in the transition to net-zero greenhouse gas emissions. To that end, there is much expectation surrounding the development of small modular reactors (SMRs), a new generation of nuclear reactors that are being marketed as the solution to all of nuclear power’s previous shortcomings.
SMRs saw an important landmark in the US last month when the Nuclear Regulatory Commission finally approved the design of a first-of-its-kind SMR from the Oregon-based nuclear firm Nuscale Power.
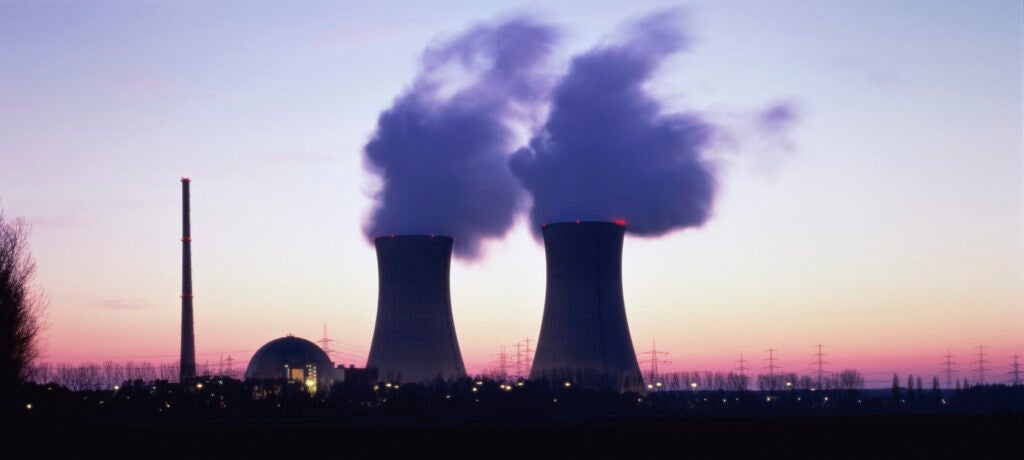
“It is a step forward on the way towards a commercial SMR, a clear signal that things are moving and that it is possible to get one licensed,” says Greg De Temmerman, managing director of the Paris-based energy think tank Zenon Research. “But every proposed SMR type will have to get licensed and, as the IEA [International Energy Agency] recently pointed out, investments in SMRs have to be stepped up now if they are to have a role in decarbonisation.”
Indeed, this giant leap for nuclear power has been promised as imminent for many a year, yet there is still no SMR in commercial operation. That begs the question: what is taking so long?
SMRs: nuclear 2.0
The International Atomic Energy Agency (IAEA) defines SMRs as advanced nuclear-fission reactors that have a power generation capacity of up to 300MW per unit – around a third of the capacity of traditional reactors. Their perceived benefits derive from the ‘small’ and ‘modular’ nature of their design. Their diminutive size means they can be sited at locations unsuited to larger nuclear power plants. Prefabricated units of SMRs could be manufactured and then shipped and installed on site, potentially making them more affordable to build than traditional reactors, which are typically custom-designed for specific places, often resulting in construction delays. The technology is designed to save on cost and construction time, and be deployed incrementally to match increasing energy demand.
The two main barriers to expanding energy access are a lack of grid infrastructure (for example, in rural areas) and the costs of grid connection (high for rural electrification). According to the IAEA, a single power plant, of any kind, should account for no more than 10% of the total installed grid capacity. Therefore, in areas lacking transmission lines and grid capacity, SMRs could be a good option to plug into the grid or run remotely off-grid to provide low-carbon power for the local population and industry. For this, microreactors – a subset of SMRs designed to generate electrical power, typically up to 10MW – would be particularly suited. They could also serve as a backup power supply in emergency situations or replace diesel-powered generators in rural locations.
How well do you really know your competitors?
Access the most comprehensive Company Profiles on the market, powered by GlobalData. Save hours of research. Gain competitive edge.
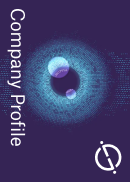
Thank you!
Your download email will arrive shortly
Not ready to buy yet? Download a free sample
We are confident about the unique quality of our Company Profiles. However, we want you to make the most beneficial decision for your business, so we offer a free sample that you can download by submitting the below form
By GlobalDataSMRs generally have simpler designs than traditional reactors and are designed to be safer by using passive systems and relying on lower power and operating pressure. The passive systems use physical phenomena such as natural circulation, convection, gravity and self-pressurisation to shut down the systems in case of an emergency, in theory meaning no human intervention is required.
They are also meant to require less fuel than traditional reactors. Power plants equipped with SMRs are designed to refuel every 3–7 years, compared to 1–2 years for conventional plants. Some SMRs are even designed to operate for up to 30 years without refuelling.
While nuclear reactors provide a consistent baseload source of clean energy, renewables such as wind and solar are variable energy sources that depend on the weather and time of day. SMRs could therefore be paired with renewable sources in a hybrid energy system to increase its efficiency.
“SMR technology is being considered with great interest by many countries as part of their energy transition towards carbon neutrality,” says Jessica Gonçalves, a spokesperson for France’s multinational electric utility EDF. “This technology is billed to replace old high CO2-emitting coal, fuel oil and gas plants around the world, and support other usages such as hydrogen production, urban and district heating or desalination.”
EDF recently announced that its NUWARD SMR reactor – currently in the conceptual design phase – will be the test case for a joint European regulatory review led by the French Safety Authority, with the participation of the Czech and Finnish safety authorities.
Across the world, public and private institutions alike are racing to bring the technology to fruition. Russia’s Akademik Lomonosov, the world’s first floating nuclear power plant, began producing energy from two 35MW SMRs in May 2020. In December last year, China Huaneng Group’s 200MW SMR at Shidao Bay began feeding power to the grid in Shandong province. Globally, there are more than 70 commercial SMR designs being developed, targeting varied outputs and different applications such as electricity, hybrid (nuclear-renewables) energy systems, heating, water desalinisation and steam for industrial applications.
“Most of the projects are in the design or licensing phases but there are two now operating in Russia and China,” says De Temmerman. “They are not commercially sold though.”
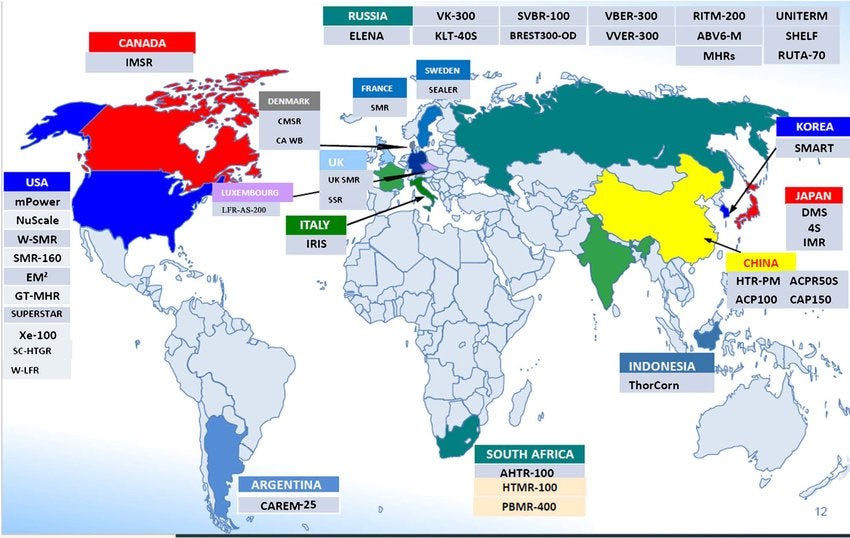
Why the holdup?
In fact, SMRs are not forecast to hit the commercial market before 2030, and although SMRs are expected to have lower up-front capital costs per reactor, their economic competitiveness is still to be proven in practice once they are deployed at scale. Nuclear reactors are extremely complex systems that must comply with stringent safety requirements, taking into account a wide variety of accident scenarios. The licensing process is extensive and country-dependent, implying some standardisation will be required for SMRs to properly take off.
“Harmonisation of regulations and requirements around the world is essential to support standardisation of design, in-factory series production and limited design adaptations to country-specific requirements,” says Gonçalves.
The protracted development time also reflects a relative lack of interest and funding in recent decades, according to De Temmerman. “Funding for nuclear is very small compared to what is invested in other energy technologies, and the recent surge in investment has largely been driven by nuclear fusion,” he says.
That said, in the US, the nuclear fission industry recently received a shot in the arm from the Inflation Reduction Act, which included several tax credits and incentives to support the continued operation of the existing nuclear fleet and the potential deployment of advanced reactors. The legislation also included $700m for the Office of Nuclear Energy to support the development of a domestic supply chain for high-assay low-enriched uranium (the fuel that SMRs will require but which is not yet available at commercial scale), and an extra $150m for the office to improve the overall R&D infrastructure at its national labs. The US Department of Energy has also identified more than 300 existing and recently retired coal plants as suitable sites for next-generation nuclear plants.
[Keep up with Energy Monitor: Subscribe to our weekly newsletter]
However – beyond the perennial oscillation of public acceptance of nuclear energy – there are still a variety of challenges SMR technology needs to overcome before it can reach commercial deployment. “The hardest is economics," says M V Ramana, the Simons Chair in Disarmament, Global and Human Security at the School of Public Policy and Global Affairs at the University of British Columbia, Canada, and author of The Power of Promise: Examining Nuclear Energy in India. "Nuclear energy is an expensive way to generate electricity.”
In the 2021 edition of its annual cost report, Wall Street firm Lazard estimated that the levelised cost of electricity (LCOE) from new nuclear plants will be $131–204 per megawatt-hour (MWh), whereas newly constructed utility-scale solar and wind plants produce electricity at somewhere between $26–50MWh. “The gap between nuclear power and renewables is large and growing,” says Ramana. “While nuclear costs have increased with time, the levelised cost of electricity for solar and wind has declined rapidly, and this is expected to continue over the coming decades.”
Even operating costs for nuclear power plants are high and many reactors have been shut down for being unprofitable. In 2018, NextEra, a large electric utility in the US, decided to shut down the Duane Arnold nuclear reactor because it estimated that replacing nuclear with wind power would “save customers nearly $300 million in energy costs, on a net present value basis”.
Those costs are for traditional nuclear power plants. SMRs, by comparison, produce relatively small amounts of electricity, but, contrary to popular opinion, Ramana thinks this is an economic disadvantage. When the power output of the reactor decreases, it generates less revenue for its utility owner, but the cost of constructing the reactor is not proportionately smaller. “SMRs will, therefore, cost more than large reactors for each megawatt of generation capacity,” says Ramana. “This makes electricity from small reactors more expensive, which is why most of the early small reactors built in the US shut down early: they just couldn’t compete economically.”
NuScale, however, claims its 12-module, 924MW plant design will have an LCOE of approximately $40–65/MWh. "NuScale’s innovative design eliminates reactor coolant pumps, large bore piping and other systems and components found in large conventional reactors, resulting in an extremely strong safety case and reduced capital and operational costs," Diane Hughes, NuScale’s vice-president of marketing and communications, told Energy Monitor.
The modular nature of SMRs’ design could also pose a safety issue, says Ramana. To combat nuclear’s unfavourable economics, SMR proposals often envisage building multiple reactors at a site, aiming to lower costs by taking advantage of common infrastructure. The configuration offered by NuScale, for example, has 12 reactor modules at each site – although it also offers four and six-unit versions. With multiple reactors, the combined radioactive inventories might be comparable to that of a large reactor.
“Multiple reactors at a site increase the risk that an accident at one unit might either induce accidents at other reactors or make it harder to take preventive actions at others,” says Ramana. “This is especially the case if the underlying reason for the accident is a common one that affects all of the reactors, such as an earthquake.” In the case of the accident at Japan’s Fukushima Daiichi plant in 2011, explosions at one reactor damaged the spent fuel pool in a nearby reactor, while radiation leaks from one unit made it difficult for emergency workers to approach the other units.
“SMRs also have a real problem with radioactive waste, because recent studies have made clear you will get a lot more of it than with large reactors,” adds Tom Burke, chairman and founding director of the environmental think tank E3G. A recent study published in the Proceedings of the National Academy of Sciences concluded that SMRs would create up to 30 times more radioactive waste per unit of electricity than conventional reactors, and that treatments to make some types of waste safe could be exploited by militants trying to obtain fissile materials.
NuScale, however, refutes the findings of the study. “[It] used outdated design information for the energy capacity of the NuScale fuel design, wrong assumptions for the material used in the reactor reflector, and incorrect assumptions on burnup of the fuel,” says Hughes. “With the correct inputs, NuScale’s design is comparable to current large pressurised water reactors on spent fuel waste created per unit of energy.”
Complementary to net zero?
Time is not on the technology’s side. The world is in a race to decarbonise. To maintain a chance of limiting warming below 1.5°C, countries must collectively reduce emissions by around 50% by 2030. It is unlikely that many SMRs will be online by then, which – although not making them redundant in the longer term – does push them down the list of development priorities against faster-deploying clean technologies like renewables and batteries.
“In Britain, we aim to decarbonise the power system by 2035, which means by 2035, we have to have affordable, reliable, non-carbon ways of meeting our electricity supply requirements,” says Burke. “If we already have those means – i.e. renewables – that can be affordable and cost competitive by 2035, why do we need nuclear that we realistically won't have widely available until after 2035?”
The role SMRs can play in the net-zero transition does split opinion, however. Although they will be late to the party, in principle the demand for non-fossil energy will be so vast it will require as many sources of generation as it can get, posits De Temmerman. The clean energy transition will be an enormous undertaking – there are more than 2,000GW of installed global coal capacity alone that must be replaced in the coming decades. On top of that, electricity production needs to vastly increase to help electrify and decarbonise other sectors such as transport. The IEA, in its Net-Zero by 2050 report, foresees nuclear capacity doubling by 2050 but keeping a constant share in electricity production, which will also need to double over the same period.
“The only role SMRs can play in having the world move towards net zero is a negative one,” argues Ramana. He points to two reasons. First, the money invested in SMRs would save far more carbon if it were invested in accelerating the switch to renewables. “There is thus an economic opportunity cost to investing in SMRs or in nuclear energy more generally,” he says. Second, building nuclear reactors is extremely time-consuming. A nuclear plant takes around a decade from the start of construction to producing electricity – and that start is often delayed by another decade to get the requisite planning and financing. “This compounds the problem of the economic opportunity cost,” says Ramana.
He contests the argument that nuclear can provide the flexibility to back up the variability of renewable energy. “If one is using a nuclear plant to back up renewables, it will be operating at an even lower efficiency," he says. "You will be spreading out a large capital cost over fewer kilowatt hours. It would be better to address the variability of renewables using a combination of demand-side responses, diversity [of technology and geography] and storage.”
De Temmerman, however, points to recent studies from Princeton University and France’s electricity transmission system operator RTE that indicate that, when considering full energy system costs, a system that includes some nuclear power would be cheaper than a fully renewable system because of the reduced overall need for storage and flexibility. “However, much depends on the assumed cost of the nuclear power, which is capex [capital expenditure] intensive; capital is now more expensive than for renewable projects.
“There are fundamental questions behind that," De Temmerman continues. "Is 100% renewables feasible? Some say yes, but there are technical challenges still to be resolved regarding flexibility and storage.”
The debate will rage on, but one thing is certain: SMRs won’t be markedly contributing to global grids any time soon.